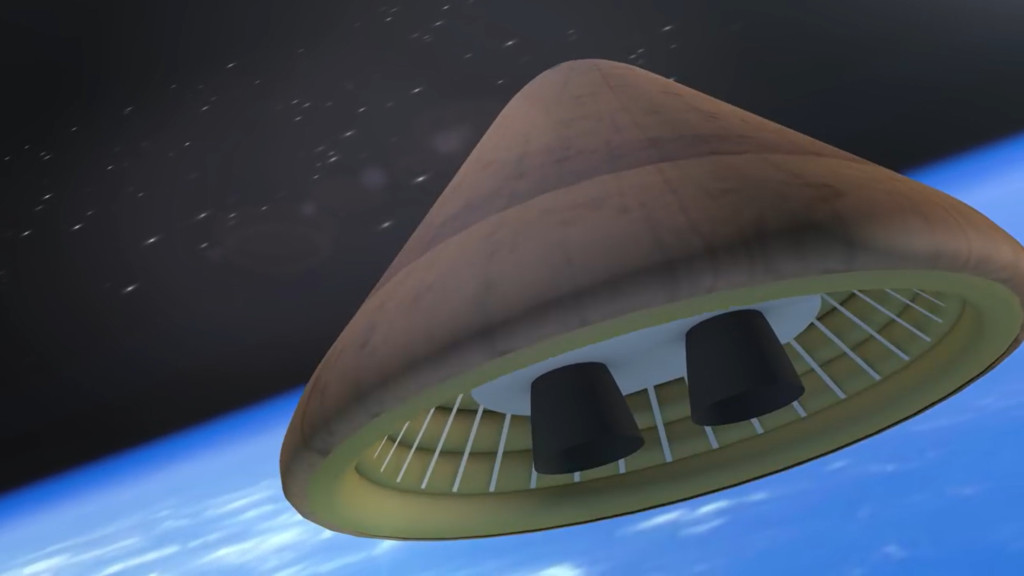
How ULA Plans To Recover & Reuse Vulcan’s BE-4 Engines
Throughout the space industry more and more companies are shifting toward either partial or full reusability. Of these companies, United Launch Alliance has always been less eager and more interested in consistent and more straightforward expendable launches. However, not long from now we will watch the company attempt to recover Vulcan’s two BE-4 engines after launch.
The SMART reuse concept was announced during the initial April 2015 unveiling of Vulcan. Here, the booster engines, avionics, and thrust structure would be detached as a module from the propellant tanks after booster engine cutoff. The module would descend through the atmosphere behind an inflatable heat shield. After parachute deployment, the two engines will land in the ocean and float on an aeroshell.
If done successfully, this process would recover both BE-4 engines which are designed for reuse. Ideally, this process would save a lot of time and money for the company and features a very reasonable breakeven point. This being said, recovering practically anything from space is no easy feat and will be a first for ULA. Here I will go more in-depth into the reuse technology, some of the possible problems, what to expect in the future, and more.
Reuse Technology
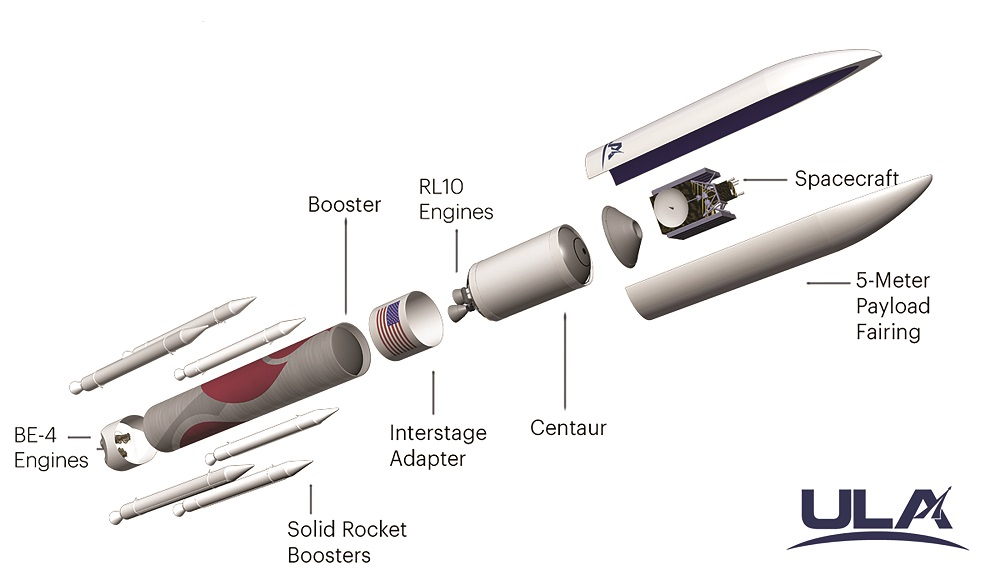
In the almost 8 years that ULA has been planning to reuse Vulcan’s engines, quite a lot of changes have been made to the recovery process. Not long ago ULA CEO Tory Bruno tweeted saying, “At the moment, component reuse (SMART) is the most economically clear return to factory approach. Upper stages that are reused should do so in space” ULA estimated this technology would reduce the cost of the first stage propulsion by 90%, and 65% of the total first-stage cost. By 2020, ULA had not announced firm plans to fund, build and test this engine-reuse concept, though in late 2019 they stated they were “still planning to eventually reuse Vulcan’s first-stage engines”
Recovery of hardware from space requires atmospheric reentry, deceleration, and landing. Reentry can be accomplished either by retro-propulsion, or by utilizing the atmosphere to decelerate the object via aerodynamic drag. Atmospheric deceleration at reentry velocities requires an aeroshell featuring a thermal protection system (TPS) to protect the payload. The aeroshell has historically been limited in diameter and area by the launch vehicle shroud. The HIAD, with its inflatable structure and flexible TPS, is an emerging technology with significant promise.
Specifically, hypersonic inflatable aerodynamic technology is applicable to entry and descent that have an atmosphere, notably Mars and our own home planet Earth. HIAD technology removes the launch vehicle shroud diameter constraint for aeroshells. A HIAD is relatively easy to pack within the launch vehicle, and can generate lift. The latter allows controllability entry via a center of gravity offset aerodynamic control surfaces like winged HIAD is inflated while still exoatmospheric slowing the reentry object from hypersonic to subsonic speeds. Years ago an initial feasibility study was performed to investigate utilizing a HIAD to recover the first-stage new Vulcan launch vehicle. It found that there is sufficient mounting and separation hardware.
After separation, the nose assembly extends to position the HIAD such that it will clear the separation plane when inflated. Saddle structures will react the majority of the drag load on the HIAD into the engine module structure. Entry simulations assuming a ballistic entry predict very high peak decelerations (15g or more). The use of a lift vector to loft the trajectory is one technique that can reduce that peak deceleration. Preliminary results indicate a 10- 12 meter HIAD will be required to recover the booster module. From here, it would continue through Earth’s atmosphere before parachute deployment. This would slow down the two BE-4s and additional technology significantly before a planned soft water landing. Here they would float on an inflatable aeroshell before being recovered by ULA and taken back to land for refurbishment.
One of the most interesting aspects of this technology and idea was a different recovery method that ULA was originally planning. Here, similar to Rocket Lab, ULA was planning to catch the bottom booster segment and two BE-4 engines with a helicopter as they parachuted down to Earth. MAR or Mid Air Recovery was developed and used extensively in the 1960s for recovery of payloads (film canisters) from space for the Corona project. In ULA’s case, they would utilize a ram-air main parachute that decelerates the payload. It would also provide a stable and predictable velocity vector that enables a helicopter equipped with a flying articulated grapple to approach from the rear and capture the in-flight parachute and gently transfer the payload mass from the parachute to the helicopter. The helicopter then transports the payload to a precise location on land or sea (e.g., barge or ship) for final recovery. In all likelihood, ULA changed this plan for something more repeatable and not as risky.
Benefits & BE-4

When asked about the economics of this recovery plan, Tory Bruno responded saying, “Breakeven in 2 to 3 flights fast” In addition, in April 2021 CEO Tory Bruno said that the additional launches purchased by Amazon for the Kuiper satellite constellation would require a higher launch cadence and that this provided support for the business case to go forward with the SMART concept. As we know a major cause of delays for ULA’s Vulcan had to do with the BE-4 engines. Blue Origin has been trying to produce the large amount necessary but was running into issues as well. This is part of the reason why this technology could be so helpful for the company. Once recovered, ULA would go through a relatively short engine refurbishment process before they are ready for the next launch.
BE-4 is one of the most powerful engines in the world and uses liquified natural gas. Blue Origin chose LNG because it is highly efficient, low cost and widely available. Unlike kerosene, LNG can be used to self-pressurize its tank. Known as autogenous repressurization, this eliminates the need for costly and complex systems that draw on Earth’s scarce helium reserves. LNG also possesses clean combustion characteristics even at low throttle, simplifying engine reuse compared to kerosene fuels. More importantly, BE-4 was designed from the beginning to be a medium-performing version of a high-performance architecture. It’s a conscious design choice made to lower development risk while attempting to meet performance, schedule, and reusability requirements. All of which helps the case for reusing BE-4 after each mission.
In terms of the launch vehicle itself, the Vulcan Centaur rocket design leverages the flight-proven success of the Delta IV and Atlas V launch vehicles while introducing new technologies and innovative features to ensure a reliable and affordable space launch service. Vulcan Centaur will service a diverse range of markets including commercial, civil, science, cargo and national security space customers. The spacecraft is encapsulated in a 5.4-m- (17.7-ft-) diameter payload fairing (PLF), a sandwich composite structure made with a vented aluminum-honeycomb core and graphite-epoxy face sheets. The bisector (two-piece shell) PLF encapsulates the spacecraft. The payload attach fitting (PAF) is a similar sandwich composite structure creating the mating interface from spacecraft to upper stage.
The Vulcan Centaur booster is 5.4 m (17.7 ft) in diameter and 33.3 m (109.2 ft) long. The booster’s tanks are structurally stable and constructed of orthogrid aluminum barrels and spun-formed aluminum domes. Vulcan Centaur booster propulsion is provided by a pair of BE-4 engines, each producing 550,000 lbs of thrust. The Vulcan Centaur vehicle is controlled by an avionics system that provides guidance, flight control and vehicle sequencing functions during the booster and Centaur phases of flight. In addition, Vulcan integrates up to six Northrop Grumman Graphite Epoxy Motor (GEM) 63XL Solid Rocket Boosters (SRBs). They are constructed out of a graphite-epoxy composite with the throttle profile designed into the propellant grain. GEM solids supported the Delta II and Delta IV rockets, and the GEM 63 variant will fly on ULA’s Atlas V rocket prior to the first Vulcan launch.
The Centaur upper stage is 5.4 m (17.7 ft) in diameter and 11.7 m (38.5 ft) long with a 120,000-lb propellant capacity. Its propellant tanks are constructed of pressure-stabilized, corrosion-resistant stainless steel. Centaur is a liquid hydrogen/liquid oxygen-fueled vehicle, with two RL10C engines. The Vulcan Centaur upgraded vehicle, flies the upgraded Centaur using RL10CX engines with fixed nozzle extensions. The cryogenic tanks are insulated with spray-on foam insulation (SOFI) to manage boil off of cryogens during flight. An aft equipment shelf provides the structural mountings for vehicle electronics. Logging an impressive record of nearly 400 successful flights and nearly 700 firings in space, RL10 engines, manufactured by Aerojet Rocketdyne, harness the power of high-energy liquid hydrogen. The RL10 boasts a precision control system and restart capability to accurately place payloads into orbit. All of which will be put to the test in only a few months on Vulcan’s maiden flight.
Conclusion
More and more companies are shifting to at least partial reusability within the space industry. What’s exciting about this process is all the unique and ambitious ideas for how the recovery of different components is completed. ULA for example is trying to recover the two BE-4 engines which are the most valuable part of the booster. We will have to wait and see how it progresses and the impact it has on the space industry.
Prompt to me please where I can read about it?